How does a NaI(Tl) detector work?
How do the electrons created in your NaI(Tl) crystal by a gamma eventually result in luminescence in the visible and ultraviolet energy band? NaI is a non-conducting crystal; this means there's a large energy separation between its filled valence band and its empty conduction band. Energetic electrons produced by a gamma interaction with the NaI material will dissipate their kinetic energy in the production of electron-hole pairs. Recombination of these electron-hole pairs can result in light emission by radiative transitions or heating in the form of lattice vibrations. The presence of thallium at the 10‑3 molar fraction level significantly increases the light emission of the crystal, and is referred to as an activator for the crystal. The radiative thallium emission is governed by an exponential law with a large decay constant. This means that luminescence light is quickly produced (few microseconds) and therefore that different scintillations event can be distinguished in time, which is important for discriminate the time of different gamma photon energy depositions.
NaI(Tl) typically converts about 11% of the incident gamma energy into photons with an average energy of 3.0 eV. The burst of photons has a characteristic (although not purely exponential) time of 0.23 ms that has a maximum emission at a wavelength of 415 nm. A 1000 keV gamma will produce, on average, 4.3 • 104 photons. Statistical variations in this number for each gamma with 1000 keV energy observed is one of the contributing factors in the observed width (energy resolution) of the photopeaks.
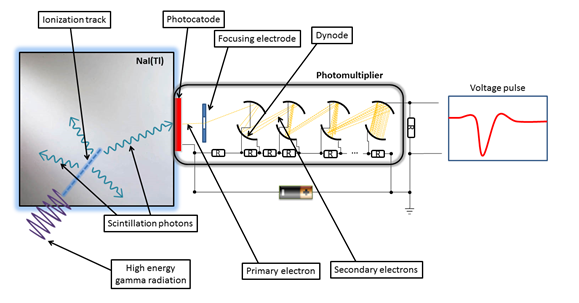
The photomultiplier tube (PMT) is coupled to the crystal in order to observe and convert into a measurable signal the scintillation light produced by the NaI(Tl). The PMT has a semitransparent photocathode converting the incident scintillation light into electrons via photoelectric effect with a certain efficiency (quantum efficiency). The PMT contains a multiplication section made up of dynodes, which are metallic plates powered by an increasingly positive voltage supply that accelerates electrons and makes them produce an avalanche, providing an amplification factor to the original primary electron signal. The primary photoelectron is therefore accelerated between the photocathode and the first dynode, striking the dynode with a kinetic energy between a few tens and a hundred eV. The collision at the surface of the first dynode produces a few electrons for each incident electron. These electrons are accelerated to the second dynode where the process is repeated for each succeeding dynode until the last electrons are collected at the anode. The multiplications of the electrons can exceed a factor of a million for typical potential differences applied across the photocathode and anode of the PMT tube. In general the multiplication factor increases with the magnitude of the applied potential difference, typically 700-1000 volts.
Up to 20% of all the photons incident on the PMT produce an electron at the photocathode. Collection efficiencies by the first dynode and the anode can change, depending upon the application and the applied potentials. The potential of each individual dynode is determined by dividing the potential difference between the photocathode and the anode with a resistor chain. This resistor chain is usually located in the detachable base of the PM tube, and can be reconfigured. The PMT is further supplied with a Multichannel Analyzer (MCA) that converts the overall pulse obtained at the end of the charge amplification stage into a digital signal, corresponding to a given acquisition channel. The acquisition channel is proportional to the gamma-ray energy as all the energy conversion mechanisms involved are proportional. Calibration with sources of known energies allows to correlate the channel number with the gamma energy and to perform the so called procedure of energy calibration.
The pulse height resolution of the detection system depends upon collecting as many of the photons created by the incident gamma-ray as possible. The photons emitted by the thallium ions are emitted in all directions, so a high efficiency reflector, Al2O3 and Teflon are used around the scintillator to reflect as much light as possible into the PMT.
NaI (Tl) detectors can be produced in different sizes: in the market it is easy to find crystals with cubic or cylindrical shapes having volumes going from 0.3 L up to 4L.
The ZaNaI detector
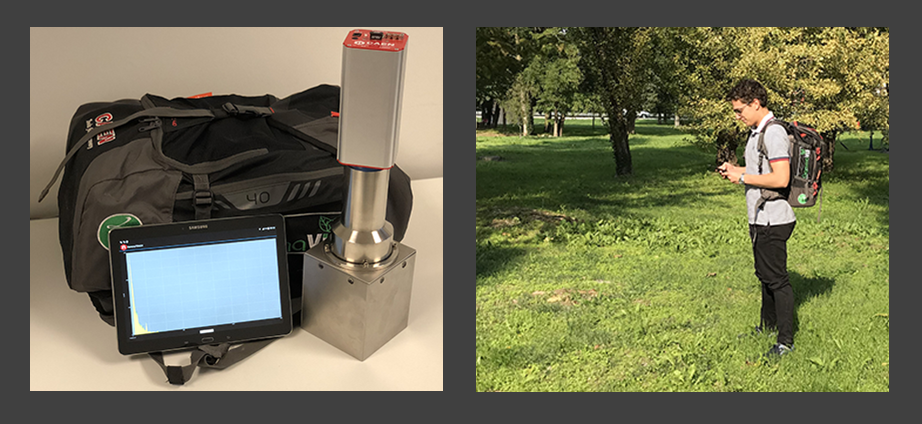
The portable scintillation gamma-ray spectrometer called ZaNaI_1L is composed by a cubic SCIONIX NaI(Tl) crystal of dimensions of 102 mm x 102 mm x 102 mm hermetically sealed in an opaque steel housing a PMT (photo-multiplier tube) base with integrated bias supply, preamplifier and digital multi-channel analyzer γStream with 2048 channels.
The energy resolution, expressed as relative Full Width at Half Maximum (FWHM) of the assembly, is of about 7.5% for the photopeak of 137Cs (662 keV). The portable scintillation gamma-ray spectrometer is placed in a backpack inside a thermo-isolator in order to protect the system from temperature changes during the in-situ measurement process.
The ZaNaI detector is managed by GammaTouch, an Android application. This application connects to the multi-channel analyzer via Bluetooth and its interface allows the operator to configure a wide range of acquisition parameters, like the acquisition duration and the voltage supplied to the PMT. The operator can also start the data-taking from the application; once started, the acquisition can be monitored in real time and the acquired spectrum is stored in the multi-channel analyzer internal memory for further processing.
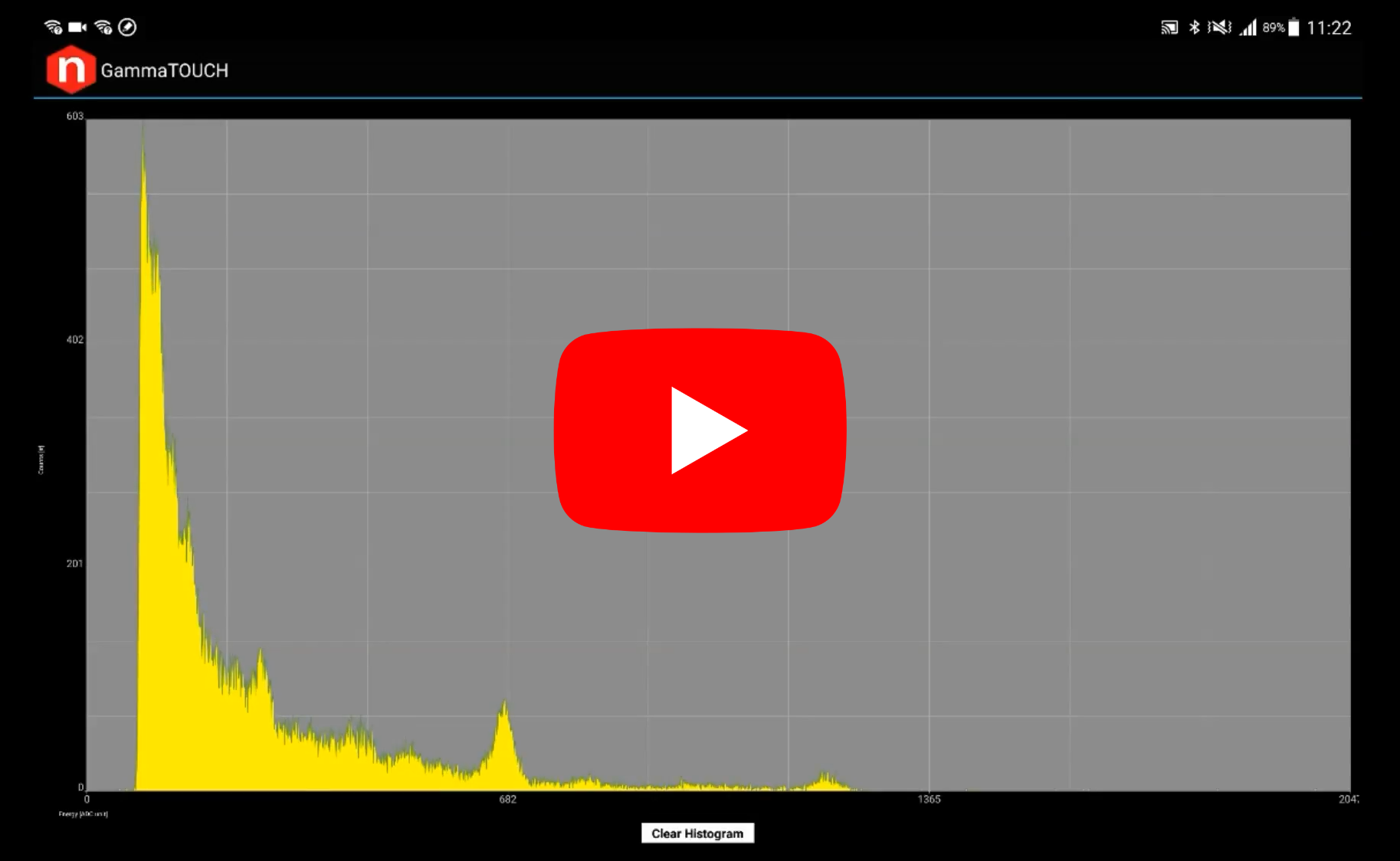
The horizontal field of view of the detector expresses the relative contribution to the total signal produced within a given radial distance from the detector vertical axis. When the ZaNaI detector is placed at an height of 0.5 m, 95% of the signal comes from a radius of ~8 m and the maximum percentage contribution to the flux comes from the concentric hollow cylinder of soil having a ~0.6 m radius centered at the detector vertical axis. When it's carried on the shoulders of the operator (1.5 m of height) the signal reaches ~95% at ~20 m and the maximum percentage contribution to the flux comes from the concentric hollow cylinder with a radius of ~1.2 m.
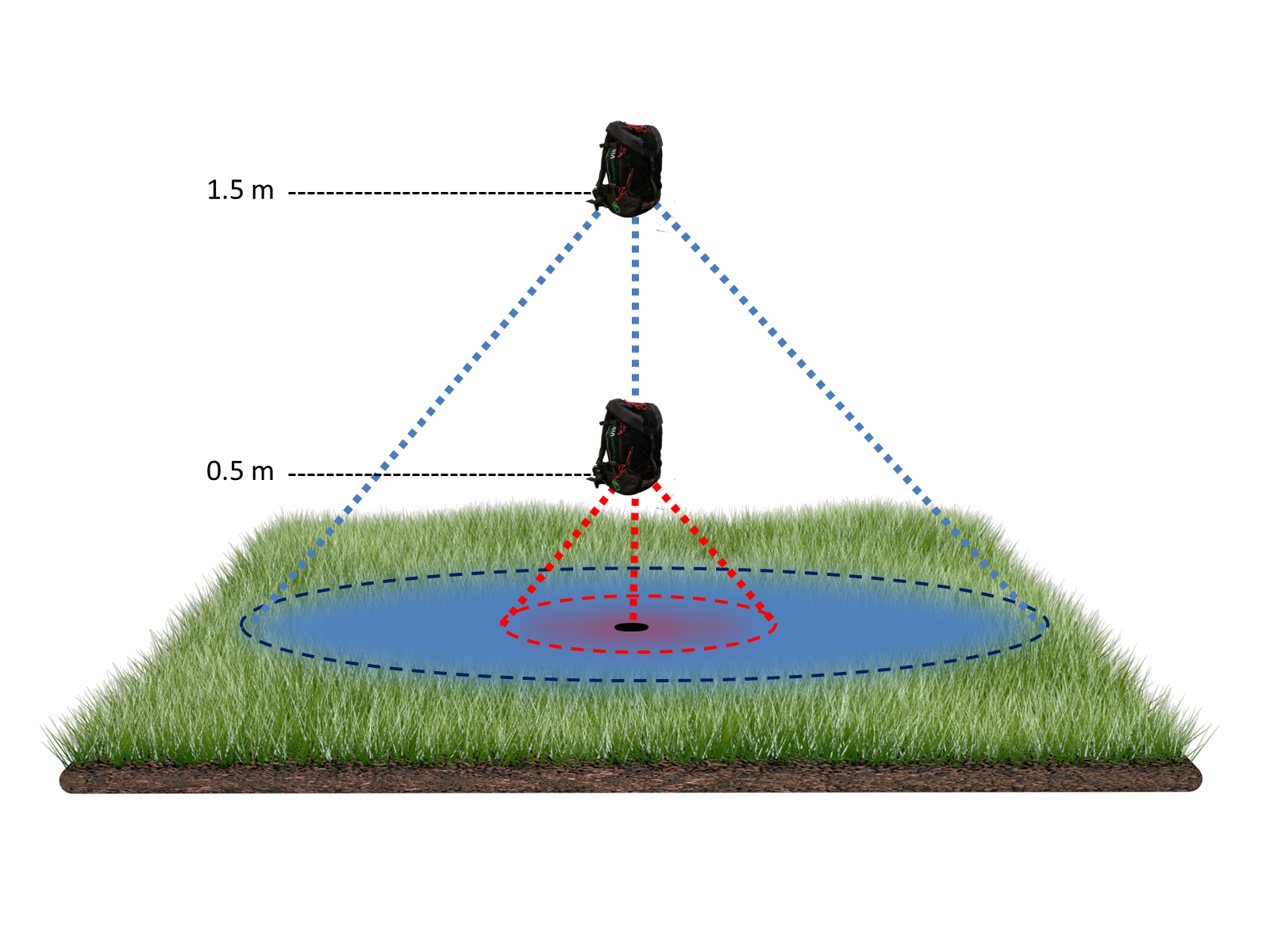
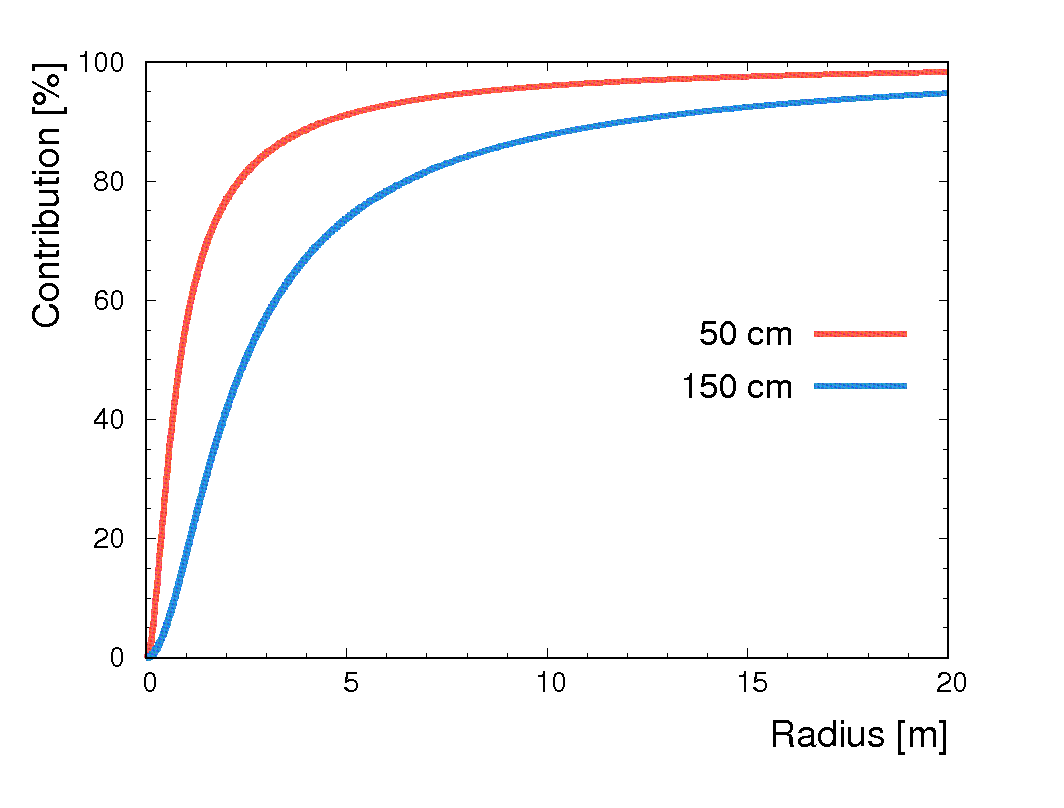
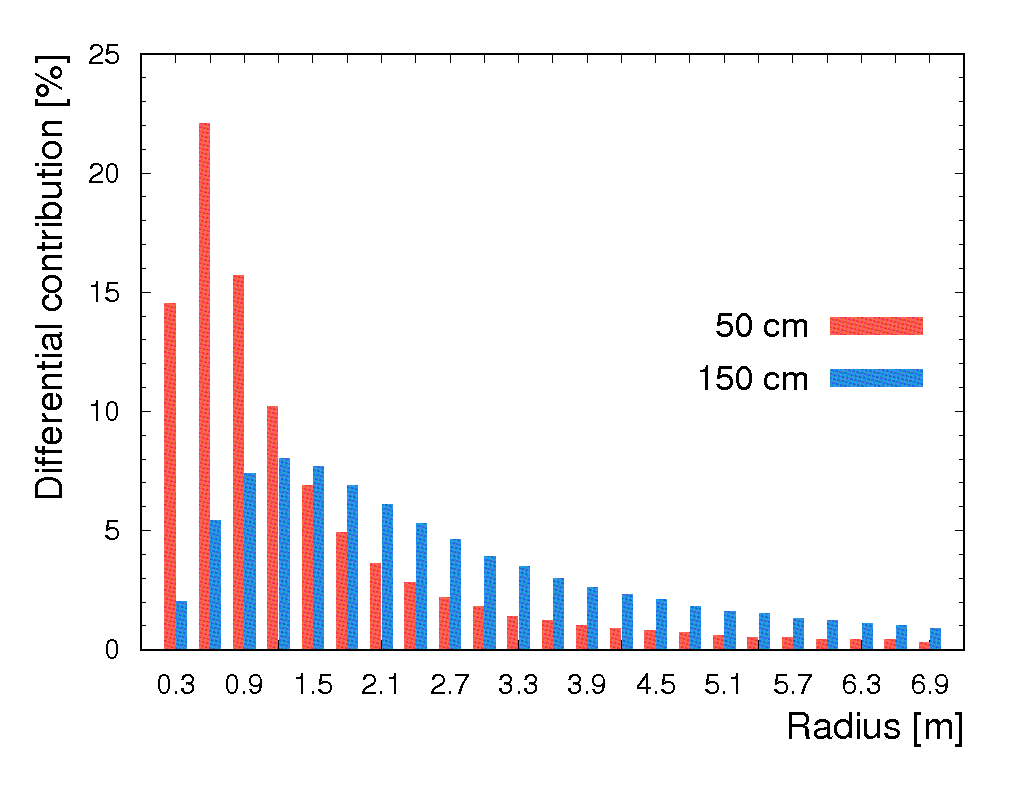