Next: Progenitor Models
Up: Theoretical Models
Previous: Theoretical Models
  Contents
The properties derived from the afterglow observations can be
explained within the framework of the relativistic fireball models,
first proposed by [Cavallo & Rees, 1978], [Goodman, 1986], [Paczynski, 1986],
in which a compact source releases
ergs within
dozens of seconds in a
Km region.
Regardless of the form of energy initially released, a quasi-thermal
equilibrium between radiation and matter is reached; this opaque
radiation-electron-positron plasma accelerates to
relativistic velocities with Lorentz factors of
.
The presence of even a small fraction of barions, around
,
makes the plasma opaque to Thomson scattering, therefore contributing
to accelerate the fireball untill a considerable fraction of the
initial energy has been converted into bulk kinetic energy.
Figure:
Cartoon for the Fireball Model.
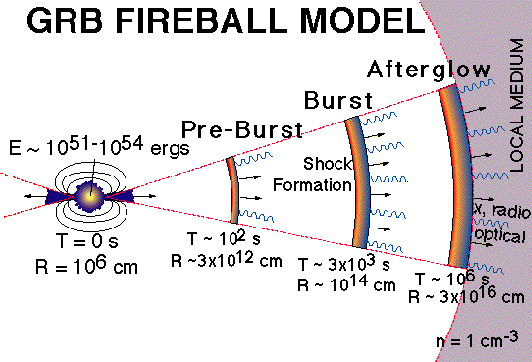 |
The extreme characteristics of GRBs seemed to lead to a paradox called
``the compactness problem'': assuming that the initial energy is released
in a volume
(
is the variability timescale,
ms),
through photons with energies distributed according to a GRB spectrum,
then the optical depth for pair production is extremely high:
.
On the other side, the obseved spectrum is definitely non-thermal;
the solution to this paradox is given by the relativistic motion for two
reasons: first, the compactness condition now becomes:
,
where
is the Lorentz factor; second, the rest frame energy of the
photons is smaller by a factor of
: therefore, only a smaller fraction
of them can create pairs. Thus, the optical depth is reduced below 1,
provided that
.
This requirement on the Lorentz factor
limits the rest mass around
the burst source, thus requiring a small baryonic contamination
(
).
Two scenarios have been proposed to convert the kinetic energy into photons:
the so-called ``internal shock'' ([Narayan et al., 1992], [Rees & Mészáros, 1994])
and ``external shock'' ([Mészáros & Rees, 1993]). According to the latter, the
relativistic matter runs into some external medium, either interstellar
or wind earlier emitted by the progenitor, while in the former scenario
the inner engine is assumed to emit many shells with different Lorentz
factors and, for this, colliding into one another, therefore thermalizing
a fraction of their kinetic energy.
The most accounted picture is the following (fig.
):
after the internal shocks produced the burst itself, the interactions
of the expanding shells with the surrounding matter are responsible for
the external shocks, which accelerate the electrons into a power law
distribution
for
.
The lower cutoff distribution is taken to be a fixed fraction of
equipartition, and a magnetic field is thought to be present behind
the shock, with a certain fraction of energy of the equipartition.
Therefore, the relativistic electrons emit synchrotron radiation,
thus providing the spectrum observed in the afterglows (for an extensive
review of the fireball model, see [Piran, 1999]).
Fig.
shows the broad band sybchrotron
spectrum in the cases of fast and slow cooling, respectively ([Sari, Piran & Narayan, 1998]).
Next: Progenitor Models
Up: Theoretical Models
Previous: Theoretical Models
  Contents
Cristiano Guidorzi
2003-07-31